The New Technologies That Could Make It Harder for Submarines to Hide
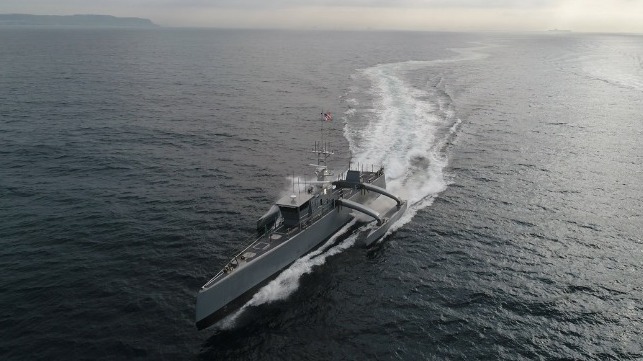
[By Sebastian Brixey-Williams]
Anti-submarine warfare (ASW) has always been a game of hide and seek, with adversarial states looking to adopt and deploy emerging technologies in submarine stealth or detection to give them the strategic edge. The advantage has shifted back and forth, but, on the whole, it has proved easier to hide a submarine than find one: the oceans are wide, deep, dark, noisy, irregular and cluttered.
Technological change can alter the balance of military power, however, and parallel technological trends facilitated by the ‘digital revolution’ may gradually make submarine detection more reliable. Certain scientific or technical breakthroughs and investments may even prove to be game-changers for submarine detection—defined here as a combination of technologies that significantly reduce or even eliminate a state’s confidence that its submarines can elude tracking and remain undetected most of the time.
History cautions that there can be no jumping to conclusions, however. Truly game-changing ASW technologies have been awaited for decades and are by nature difficult to predict. This was clearly expressed in Western deterrence and arms-control literature in the 1970s and 1980s, which reflected fits of ‘transparent oceans anxiety’: a persistent and partially unfalsifiable disquiet that a technological innovation could make the oceans transparent and undermine strategic stability by making US nuclear-powered ballistic missile submarines (SSBNs) sitting ducks in a bolt-from-the-blue first-strike attack. Technologies available towards the end of the Cold War were insufficient to give seekers the advantage that some analysts predicted and, as Owen Cote notes, also contributed directly to the development of effective countermeasures that ensured the survivability of US SSBNs. After the Cold War, the notion that submarines (above all, SSBNs) were ‘invisible’ became politically unassailable.
Several articles and studies in recent years have revisited the survivability of SSBNs, for which game-changers would perhaps have the greatest consequences for international security. As Norman Friedman notes, ‘strategic submarines seem to be key to strategic stability’, providing what is generally believed to be the most survivable nuclear second-strike force. Friedman marshals some of the limited evidence available in the public sphere, but is deliberately cautious about making bold and certain predictions.
The technologies outlined here relate primarily to emerging ASW capabilities developed by the US, which has higher levels of transparency about its SSBN capabilities and nuclear strategy than other countries, but it may be assumed that similar technologies will proliferate to other navies.
Sensor platforms
ASW traditionally relies on a limited number of costly manned platforms such as attack submarines (SSNs and SSKs), frigates and maritime patrol aircraft fitted with a variety of sensors. Today, there’s evidence of a move away from this model towards unmanned aerial vehicles (UAVs), unmanned surface vehicles (USVs), and unmanned underwater vehicles (UUVs) fitted with equivalent sensors, which are more expendable and are becoming cheaper to develop, produce, modify and deploy at scale. Navies are indicating that this is the direction of travel; as Robert Brizzolara, a US Office of Naval Research program officer, states: ‘The U.S. military has talked about the strategic importance of replacing “king” and “queen” pieces on the maritime chessboard with lots of “pawns”.’
A prime example is the US Navy’s medium displacement USV, or MDUSV. The prototype launched in April 2016, Sea Hunter, was reported to have demonstrated autonomous SSK detection and tracking from the ocean surface from 3.2 kilometers away, requiring only sparse remote supervisory control for patrols of three months, using a combination of ‘advanced hydro-acoustics, pattern recognition and algorithms’. Since the range and resolution of acoustic sensors are highly variable according to oceanic conditions (such as depth, temperature and salinity), the range may well go further in favorable conditions; a Chinese estimate puts it at 18 kilometers. Since SSKs using air-independent propulsion or running on batteries are virtually silent, MDUSVs should theoretically be capable of pursuing SSNs and SSBNs (whose nuclear reactors continuously emit noise) at greater distances, and there are reports that they will be armed.
Whereas the new US FFG(X) frigate costs a sizeable $1 billion per ship, MDUSV platforms are reported to cost only $20 million each and so could conceivably be produced at scale to autonomously or semi-autonomously seek and trail submarines. Former US deputy defence secretary Robert Work has suggested as much: ‘These will be everywhere.’
Signal processing
ASW relies on separating tiny submarine signals from background ocean noise, primarily by using active and passive acoustic sensing (sonar) and magnetic anomaly detection (MAD), and it looks likely that these will remain the most important signals in the near future. However, the range of signals may grow as sensor resolution, processing power and machine autonomy reach the necessary thresholds to reliably separate other, ‘quieter’ kinds of signal. As Bryan Clark notes, ‘While the physics behind most [non-acoustic detection] techniques has been known for decades, they have not been exploitable until very recently because computer processors were too slow to run the detailed models needed to see small changes in the environment caused by a quiet submarine.’ However, he adds there’s now ‘the capability to run sophisticated oceanographic models in real time’.
No breakthroughs have been publicly disclosed, though an independent investigation by British Pugwash in 2016 identified light detection and ranging, or LIDAR, using blue–green lasers; anti-neutrino detection; and satellite wake detection as signal types that may merit further examination. Higher processing power can also enable digital sensor fusion, whereby different kinds of signal are synthesized and analyzed together, and better simulations of the baseline ocean environment, which would show up anomalies in greater contrast.
Persistent observation
Tracking submarines across large areas of ocean remains a key challenge for ASW. Manned platforms have limited ranges, and while the US Navy’s passive sonar system, SOSUS, is still in operation in parts, it is geographically bounded and requires substantial modernization to detect today’s quiet submarines. This gap has been partially filled by modern acoustic sensor arrays like the fixed reliable acoustic path, but in relative terms these cover very small areas of ocean.
Distributed remote sensing networks, however, which link interoperable manned and unmanned sensor platforms together as nodes in a larger system of systems, could be used to scale up persistent observation across wider areas. Networks in development include the US Defense Advanced Research Projects Agency (DARPA) distributed agile submarine hunting program, which is developing ‘a scalable number of collaborative sensor platforms to detect and track submarines over large areas’, and PLUSNet (persistent littoral undersea surveillance network), which aims to create ‘a semi-autonomous controlled network of fixed bottom and mobile sensors, potentially mounted on intelligent [unmanned platforms]’ in littoral zones.
Networks of this type could be greater than the sum of their parts, with nodes able to carry heterogeneous sensors, cross-reference positive signals from multiple directions and domains, and move and respond to get a better look at signals using real-time swarming. It’s easy to imagine fleets of MDUSVs being used in the same way, potentially much further apart. Some technical challenges remain, including scaling up to blue water and improving underwater communication, autonomous decision-making, self-location and battery life, but none appear insurmountable and some of the physical limitations felt by a single vehicle can be mitigated by swarming.
Sensor resolution
While it seems likely that the proliferation of distributed remote sensing networks could decrease the importance of extending sensor range and resolution as the quantity of platforms goes up, the two principal ASW sensor types (sonar and MAD) have, or are hoped to enjoy, significant improvements in resolution on their Cold War antecedents.
Acoustic sensing in peacetime relies mostly on passive sonar, as active sonar ‘pings’ of adversary submarines risk a hostile response and disrupt ocean fauna. Recent techniques under development at the Massachusetts Institute of Technology’s Laboratory for Undersea Remote Sensing, which use particular features of the ocean as acoustic waveguides for efficient long-range propagation, offer the potential for significantly greater ranges to detect and classify submarines under certain conditions. The POAWRS (passive ocean acoustic waveguide remote sensing) system was able to ‘detect, localize and classify vocalising [marine mammals] from multiple species instantaneously’ over a region of approximately 100,000 square kilometers, and detect quiet diesel-electric surface vessels ‘over areas spanning roughly 200 kilometers in diameter’ (30,000 square kilometers). The active variant (OAWRS) can localize manmade objects as short as 10 metres over areas 100 kilometers in diameter (8,000 square kilometers), provided the resonant frequencies scattered by the object are known. Crucially, by using many frequencies transmitted at once—multi-frequency measurements—the system can distinguish fish or seafloor clutter from manmade targets. POAWRS can also be mounted on unmanned vehicles and used to detect larger manmade objects like submarines, even if their signal is partially mitigated by acoustic cloaking.
Today’s MAD magnetometers can detect a submarine’s ferromagnetic hull at a maximum range of several hundred meters. The use of more sensitive magnetometers with a range around an order of magnitude higher, known as superconducting quantum interference devices, or SQUIDs, has been limited by their oversensitivity to background noise and their need for super-cooling.
However, in June 2017, an announcement by the Chinese Academy of Sciences, which was later taken down, claimed that a Chinese team had produced a ‘superconductive magnetic anomaly detection array’, which technical experts indicated could have ASW applications and could contribute to a wider strategy to create a ‘Great Underwater Wall’ to monitor underwater traffic in and out of the South China Sea. One expert in magnets estimated that such an array could have a range of 6 kilometers or further. If this technology can be proved to work and be mounted on unmanned platforms, it could have significant implications for shorter-range submarine detection, though these reports remain unverified in the public domain.
Data transmission speed
Most data can be transmitted in ‘nearly real time’ through air. Undersea communications are more challenging, as radio waves are heavily absorbed by water. While acoustic signals can be used, this has remained an expensive technique involving significant processing power. As a workaround, DARPA’s POSYDON (positioning system for deep ocean navigation) program looks to relay data between UUVs via low-frequency acoustic messages to USVs, and from them by radio to satellites, which can make use of radio waves.
Meanwhile, a team at Newcastle University in the UK has developed ultra-low-cost acoustic ‘nanomodems’, which can send data via sound up to two kilometers for use in short-range underwater networks. Improving the ‘intelligence’ of each node in the network so it can discriminate useful data and minimize data packets would also increase the speed of transmission.
Hurdles still remain, but it seems that low-cost workarounds can be found.
Conclusion
The introduction of autonomous, unmanned platforms mounted with improving and digitally fused sensors, integrated within cooperative systems, will enable wider surveillance of the ocean. One effect may be to elevate the reliability of submarine detection and, in some circumstances, these technologies could prove to be game-changers that tip the balance in the favour of ASW. Nevertheless, because the history of science and technology is littered with unforeseen obstacles and elusive breakthroughs, and because many of these technologies are currently classified, it’s difficult to offer any kind of firm timeline for game-changers in ASW.
According to James Clay Moltz at the US Naval Postgraduate School, writing in 2012, some ‘emerging autonomous-tracking technologies … are likely to be widely available within the next 20 years … [raising] the prospects for successful ASW against US forces’. If this proves correct, in spite of the United States’ world-leading stealth technologies, it would imply that nuclear-capable states in the Indo-Pacific deploying relatively noisy SSBNs might have even weaker prospects of survival by the early 2030s. This would have important implications for India’s first-generation Arihant-class SSBN and China’s Type 094, for example.
As the technological picture becomes clearer, future work will need to continually evaluate the relative gains and losses in detection and survivability that these technologies could provide to each state, and offer tangible responses to reduce strategic nuclear risks both in the region and globally.
Sebastian Brixey-Williams is co-director of the British American Security Information Council (BASIC) and a nuclear weapons expert with a special focus on nuclear responsibilities, emerging technologies, risk reduction and disarmament politics.
This piece was produced as part of the ?Indo-Pacific Strategy: Undersea Deterrence Project, undertaken by the? ANU National Security College. This article is a modified version of chapter 19, ‘Prospects for game-changers in detection technology’, published in the 2020 edited volume? The future of the undersea deterrent: a global survey. Support for this project was provided by a grant from Carnegie Corporation of New York.
This article appears courtesy of The Strategist and may be found in its original form here.
The opinions expressed herein are the author's and not necessarily those of The Maritime Executive.