Why the U.S. Navy Needs to Get Back to Prototyping
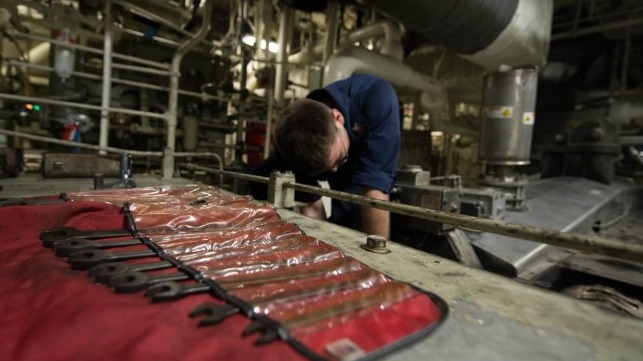
By John Hanley
Broad agreement exists that the Department of Defense’s, and thus the Navy’s, acquisition system is bound like Gulliver by Lilliputian processes, resulting in an inability to adapt. This inflexibility threatens to increase the risks to operating forces as they face a growing number of adaptive adversaries, ranging from China and Russia, North Korea and Iran, to the Islamic State, Al Qaeda, and others. Well-intended legislation and increasing reliance upon computer modeling to inform the selection of future platforms and systems are major contributors to the current situation. Greater reliance on experimenting with prototypes at sea could provide a large improvement.
Introduction
Congress passed the Goldwater-Nichols legislation in 1986 to promote joint operations and provide more civilian control by creating an Undersecretary of Defense for Acquisition and reducing the role of the Chief of Naval Operations (CNO) and other Service Chiefs in acquisition decisions. This legislation added joint duty requirements to the already-packed career paths for line officers, even as it added new educational and experience requirements for acquisition professionals. The Defense Acquisition Workforce Improvement Act in 1990 further created mandatory requirements for a more professional acquisition force. Line and acquisition professionals “had completely different chains of command and, consequently, were situated in different performance evaluation and promotion structures.” Having little appreciation for an increasingly complex acquisition process, line officers had trouble articulating their needs to an acquisition workforce that was itself increasingly isolated from the operational environment.
Though the Packard Commission that informed Goldwater-Nichols legislation called for more prototyping to gain experience with new platforms and systems before making major investments, the Department of Defense (DoD) and the Navy increasingly turned to computer-based combat and campaign simulations as a cheaper and more flexible way to inform acquisition decisions. This had the effects of further separating the experience of fleet operators from Navy acquisition, and removed an important source of data for ensuring computer-based simulations were accurate.
In their book Switch: How to Change Things When Change Is Hard, Chip and Dan Heath highlight the value of bright spots; examples of projects that work well to make a case for needed change. This article suggests some bright spots, and continuing challenges, in acquiring capabilities the Navy needs to adapt to rapidly emerging security opportunities and challenges.
A Virtuous Prototype Cycle
As a junior officer, I was privileged to be assigned to the USS Guitarro (SSN 665) in San Diego in 1973. The Guitarro played a major role in developing tactics for prototype combat systems deployed to the Pacific submarine fleet, in particular the new Submarine Towed Array Sensor System (STASS) along with its BQR-20 series digital sonar displays. In the mid-1970s, Guitarro also installed the first digital submarine combat system (BQQ-5 sonar and Mk-117 fire control system) and participated in the development of submarine-launched Harpoon and Tomahawk cruise missiles.
Following my service on the Guitarro, I became an operations analyst supporting several programs. The Naval Electronics Systems Command (PME-108) was sponsoring the Coordination in Direct Support (CIDS) program developing technology and techniques for communicating with submarines to operate in direct support of carrier battle groups, and the Over-the-Horizon Targeting (OTH-T) program was developing technology and techniques for targeting ships with Harpoon and Tomahawk missiles at ranges beyond the line of sight. These programs integrated their efforts with the Tactical Development and Evaluation Program sponsored by the OP-953 on the Navy staff. My next job involved working with the Chief of Naval Operations Strategic Studies Group where I witnessed the speed with which a small team of intelligence specialists, engineers using the latest technology, and Navy leadership could deliver cutting edge capabilities to the fleet very rapidly.
My experience in these programs taught the value of providing prototypes to the fleet early. Working with prototypes allowed us to develop tactics and techniques that the system developers never considered, and highlighted operational limitations and misperceptions of those developing the systems. Fleet analysis data contributed directly into operations analysis, computer simulations, and war games. The experience also demonstrated the limitations of tightly-coupled integrated systems as opposed to systems with modules that could adapt and change easily. As my career continued, I observed revisions to the DoD acquisition system that diminished the role of prototyping and extended times to demonstrate new capabilities to the fleet, usually exceeding cost estimates and requiring modifications as operators discovered what they could, and could not do.
Sonar Towed Arrays and Digital Displays
STASS was a long, linear array of hydrophones deployed behind the submarine on a cable. This kept the array’s sensors away from the towing submarine’s radiated noise, significantly improving the signal-to-noise ratio needed to detect faint signals. It could detect contacts behind the submarine that were screened from the hull-mounted sensors in the bow. Its length provided a larger aperture to detect lower frequencies at longer ranges. This sonar system made submarines more effective.
However, the new system had its challenges. Initially, a sonar operator could monitor only one of the array’s 16 beams at a time, by listening and/or monitoring the BQR-20’s digital display.9 The display would provide a waterfall of illumination if a signal was detected on that beam. Low frequencies required several minutes of integration time to process signals from the ambient noise. Thus it could take more than an hour to search though all of the beams. The submarine also had to travel at slow speed to prevent the noise from water flowing over the hydrophones from masking signals from other vessels. Even with the slower speeds, the longer detection ranges provided the new sonar system significantly increased the search rate in deep ocean areas.
The principal tactic for estimating a targets range using passive sonar was developed by Lieutenant John Ekelund in 1956.10 Ekelund’s approach significantly improved upon target motion analysis techniques that involved only plotting bearings to a target over time. His method involved calculating the rate of change of the relative bearing of the contact as the host submarine maneuvered on two courses. The time to do the calculation affected the accuracy of the estimate. Slow maneuvering with the STASS was frustrating.
Our sister ship, USS Drum (SSN 677), was the first ship in the Pacific fleet to receive the new STASS. To reduce the time maneuver to a new course, Drum tried a tactic of speeding up through the turn, then slowing to reduce the flow noise. Unfortunately, the sub slowed faster than the array, resulting in the array’s cable wrapping around the horizontal stabilizer on the sub.
Guitarro then had its opportunity to develop tactics for employing the STASS. Our efforts focused on three areas: maneuvering the ship, sonar search procedures, and plotting contacts. I had the lead on plotting. Current practice used a “compressed” time-bearing plot along with “strip” plots. The time bearing plot provided bearing rates needed to compute Ekelund ranges. Speed strips marked with various speeds were manually aligned across bearings to a contact’s for estimating its range, course, and speed. Given the time required to generate contact bearings with the STASS, we developed an “expanded” time-bearing plot.
A big innovation occurred when Dr. Ted Molligen (a ship rider from Analysis and Technology, Inc.) noted that the array’s beams were cones and the sea bottom was a plane. The intersection of a cone and a plane is a hyperbola. Therefore, when the contact’s signal bounced off the bottom, which occurred frequently in the Pacific, we were dealing with lines of bearing along a hyperbola. Within a day, we manufactured templates of hyperbolas out of plexiglass for strip plotting using bottom bounce signals. Without measuring bearing rates, the intersection of two hyperbolas provided a contact’s estimated position quickly after our maneuver.
Another unanticipated effect was the ability to observe the contact’s Doppler signal shift in near-real time. Thus we could observe not only the contact’s bearing change during maneuvers, but also whether it was opening or closing us. Reconstructed plots of our target clearing its baffles (simulating “crazy Ivans”) during exercises showed our depiction of the target’s motion to be very accurate.
The next breakthrough occurred when we received the BQR-22 a couple of months later. The BQR-22 could process two beams simultaneously. We discovered that, with some regularity, we would receive both direct path and bottom bounce signals from the contact. The different signals would arrive on different beams because of their paths through the water. The intersection of a direct path line of bearing with a bottom bounce hyperbola produced an estimate of the target’s range without having to maneuver. Exercise reconstruction showed our estimates to be within a few percent of the target’s range.
Under the leadership of our superb Executive Officer, Lieutenant Commander Dan Bacon, we documented the tactics we had developed for maneuvering the sub, conducting the sonar searches, and plotting in a tactical memo and submitted it to Commander, Submarine Forces Pacific. He replaced our cover with his, and distributed it as a Tactical Memorandum to the fleet.
Within a year, we received the BQR-23 that processed four beams simultaneously. We then deployed with this sonar system, and other prototype sensors and processors, for operations in the western Pacific. Deploying with prototype equipment was routine in the submarine force.
During World War II U.S. submarines could attack only surfaced enemy submarines.11 In 1949, the submarine force created Submarine Development Group 2 and tasked it with antisubmarine warfare (ASW) as part of an effort to preserve the submarine force structure during demobilization. Within twenty years, the U.S. submarine force went from having essentially no ASW capability to becoming the dominant ASW force in the world. Following their motto of “Science, Technology, Tactics”, the Group employed a program of designing, conducting, and reconstructing exercises to develop tactics for prototype systems, and reconstructing submarine performance during operations using extensive data collected during patrols.12 Using the Group’s methodology, we were able to exploit the STASS and the BQR-20 series digital displays and document proven tactics for the fleet that significantly improved the U.S. advantage over Soviet submarine forces within an 18 month period.
In contrast, installing the first submarine digital combat system in the shipyard demonstrated challenges that occur when developing systems without prototyping. The system had no feature for entering bearings directly from the periscope. Apparently, the engineers thought that all approach and attack would use sonar only. We also were told that adding hyperbolic ranging to the software in the central computer complex, which serviced the sonar and fire control system, would take at least a decade. Stand-alone computers came to support search planning and target motion analysis since the integrated system was incapable of rapid change.
Coordination in Direct Support
Admiral Rickover had pushed through the development of the Los Angeles-class submarines by arguing that their higher speed would allow them to screen a carrier battle group. The major problems were communicating with submarines to keep them on station as the battle group maneuvered, to direct them to prosecute contacts detected by other battle group platforms, and to prevent other battle group ASW forces from attacking them. Also, based on the way that the U.S. targeted German U-boat radio transmissions during World War II, our silent service routinely disabled its radio transmitters while on patrol to prevent detection. Standard submarine communications involved the submarine getting an antenna to the surface for broadcasts that were repeated for eight hours on a two-hour cycle. The submarine restricted its speed to a few knots when at communications depth, both to prevent anyone seeing the wake of the periscope and to keep its floating wire antenna on the surface. Thus the submarine could best communicate at scheduled intervals, and could not transit at battle group speeds while communicating.
Rear Admiral Guy H.B. Shaffer took the methods he had used commanding Submarine Development Group 2 with him to the Naval Electronic Systems Commands program office PME-108. He established the Coordination in Direct Support (CIDS) program to develop means to communicate with submarines providing direct support to carrier battle groups.
The Submarine Analysis Notebook provided the methodology and data required for assessing submarine ASW performance. The first step in the CIDS program was to develop a Fleet Exercise Analysis Guide that provided a conceptual battle group ASW process and performance metrics.15 PME-108 then worked with the Tactical Development and Evaluation (TAC D&E) Program and the numbered fleets to schedule participation in their exercises, and invited the Navy laboratories to provide prototype communications systems for submarine communications. The prototypes included everything in the electromagnetic spectrum from blue-green lasers to Extremely Low Frequency (ELF) radios and a variety of acoustic communication methods. For each exercise a team would work with relevant commands to design the exercise and develop data collection plans. The team would then ride key ships in the exercise providing advice on accomplishing exercise training and tactical development objectives, and overseeing the data collection. Following the exercise, the team would reconstruct and analyze the event in full, including documenting the timelines for each ASW interaction and every ASW communication over every communications path.
This approach allowed prototypes to be evaluated not just as stand-alone systems, but demonstrated their value both in enhancing communications as part of a suite of systems operating simultaneously and in accomplishing the mission of protecting the carrier from submarines attacking with torpedoes and cruise missiles.
Occasionally a laboratory would offer a prototype that was operationally unsuitable. One such system was a shaped buoy weighing several thousand pounds to be towed behind a submarine at depth and speed to push an antenna to the surface. Had the buoy hit a surface vessel, or submarine at shallower depth, it would have had the impact of a torpedo without the explosion.
Documenting every step of the communications path demonstrated the delays created by communications controlled by the submarine operating authority ashore. This led the submarine force to provide Submarine Element Coordinators (SEC) at sea with the battle group. The exercises explored many operational schemes with these SECs adjusting submarine broadcast schedules and using ELF or acoustic “bell-ringers” to call the submarine to communications depth for higher data rate communications.
After 10 fleet exercises conducted over a three-year period involving all the numbered fleets, the CIDS program demonstrated that the tactical concept for using submarines as an outer screen moving with the carrier battle group was infeasible. This led to alternative schemes for employing submarines supporting task groups. The communications data proved valuable and was incorporated in the Navy’s Warfare Environment Simulator which allowed teams playing task group platforms on different terminals to receive information with realistic time delays.17 Over time, this became the Navy Simulation System, but lost its original purpose of focusing on command and control issues using fleet data.
Over-the-Horizon Targeting
Shortly after the command and control fleet exercises, the Navy began deploying Harpoon and was getting ready to deploy Tomahawk missiles to the fleet. So RADM Shaffer established an Over-the-Horizon Targeting (OTH-T) program within PME-108. The approach followed the CIDS program; developing a fleet exercise analysis guide, designing exercises to incorporate prototype systems and tactics, collecting data, and conducting analyses. The Mediterranean, with its high shipping density and many islands, provided the most challenging environment for OTH-T.
The exercises were again successful in demonstrating that the technology and tactics were insufficient to support the proposed concepts for anti-ship Tomahawk use. This and the abundance of targets ashore were major factors in emphasizing land attack versus anti-ship versions of the Tomahawk missile.
Advanced Technology Panel
By the late 1970s, Navy efforts to develop special intelligence sources provided deep penetration of Soviet Navy thinking and practices. The CNO repurposed the Navy’s Advanced Technology Panel (ATP), created in the 1970s, to become the main customer for this highly restricted intelligence. The ATP was a small group of the senior admirals on his staff, his top ‘thinkers’, who were cleared primarily to review special programs, but did a lot more. Working closely with the Navy laboratories, the leadership could deliver counters to what the Soviets were deploying within months to a year or two of having firm intelligence on their systems.
CNO Admiral Tom Hayward, on the advice of then Under Secretary of the Navy Robert Murray, formed a Strategic Studies Group of six promising Navy officers selected personally by him and two Marines at the Naval War College in 1981. Murray characterized the SSG as changing captains of ships into captains of war, employing terms that Winston Churchill used when he said that he needed more of those in World War I.
That fall, the ATP led by Vice CNO Admiral Bill Small was looking for ways to game using new, sensitive intelligence. In January 1982, the SSG was asked to develop concepts employing the new intelligence. The SSG held an extensive war game in April 1982. Admiral Small brought the ATP to Newport for two days at the conclusion of the game to review the results. The concepts used in the game became the foundations for the 1980s Maritime Strategy and rapidly changing war plans. The ATP was able to focus special programs on providing capabilities tailored to executing the new war plans.
Two Different Paths: Nuclear Submarines and Distributed Surface Combat Power
Prototyping should not be restricted only to the payloads on vessels. In 1951, then Captain Hyman G. Rickover received authorization to build nuclear powered submarines. USS Nautilus (SSN 571) was commissioned in 1954 with a pressurized water reactor. The Navy then commissioned:
- The USS Seawolf (SSN 575) with a liquid metal cooled reactor in 1957. This design presented too many risks and was quickly replaced.
- The USS Triton (SSRN 586) in 1959, a large radar picket submarine with two reactors.
- The USS Tullibee (SSN 597) in 1960, a very small, quiet submarine with a small reactor.
- The USS Jack (SSN 605) in 1967 with direct drive and counter-rotating shafts and propellers.
These submarines, along with the small classes of SSNs built between the prototypes, explored the design space, adapted design features, and informed the building the following classes of nuclear submarines. The large capacity of the USS Halibut (SSGN 587), designed to shoot Regulus nuclear cruise missiles, allowed it to adapt to different missions over its service life.
In 1996, the CNO Strategic Studies Group briefed its concepts for dispersed and distributed surface power to the CNO. The Group had in mind fast, stealthy ships of several hundred tons capable of mounting modular payloads for different missions. They anticipated that the Navy would explore the design space with prototypes, as it did with nuclear submarines. Instead, DoD acquisition processes led to the Littoral Combatant Ship. Rather than using a range of small and large prototypes using differing propulsion concepts, the Navy ended up with two much larger ship classes that have had many early difficulties.
Conclusion
The DoD acquisition system has come to believe that we must precisely predict the threat decades into the future, optimize designs by spending many million dollars on computer analysis, and then commit billions of dollars for procurement, without any of the experience and operator feedback provided by prototypes. This developmental approach incurs major cost, schedule, and performance risks because the future remains stubbornly uncertain – just as it always has been.
A better alternative is to prototype operational systems and platforms rapidly, providing agility to adapt to emerging threats and take advantage of emerging technology. Programming, budgeting, and contracting processes present major hurdles. Though routine acquisition procedures do not support such agility, Other Transaction Authority and similar processes authorized by Congress should be employed to their maximum extent. However, to do so effectively will require reinvigorating experimenting with prototypes in fleet exercises in ways similar to Submarine Development Group 2, the CIDS and OTH-T programs, and early nuclear submarine force development.
Captain John T. Hanley, Jr., USNR (Ret.) began his career in nuclear submarines in 1972. He served with the CNO Strategic Studies Group for 17 years as an analyst and Program/Deputy Director. From there in 1998 he went on to serve as Special Assistant to Commander-in-Chief U.S. Forces Pacific, at the Institute for Defense Analyses, and in several senior positions in the Office of the Secretary of Defense working on force transformation, acquisition concepts, and strategy. He received A.B. and M.S. degrees in Engineering Science from Dartmouth College and his Ph.D. in Operations Research and Management Sciences from Yale. He wishes that his Surface Warfare Officer son was benefiting from concepts proposed for naval warfare innovation decades ago. The opinions expressed here are the author’s own, and do not reflect the positions of the Department of Defense, the US Navy, or his institution.
This article appears courtesy of CIMSEC and may be found in its original form here.
The opinions expressed herein are the author's and not necessarily those of The Maritime Executive.