Re-Introducing Steam Power into Commercial Maritime Propulsion
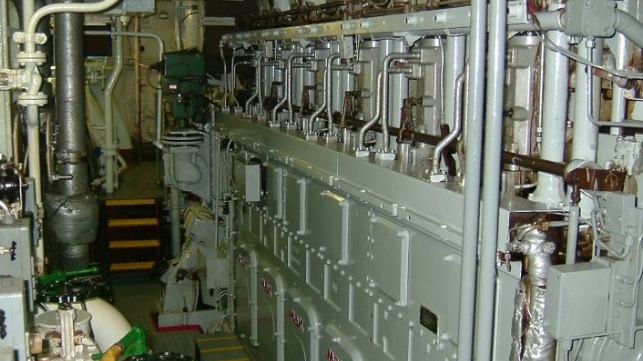
While naval vessels have traditionally used combined-cycle gas-turbine-and-steam propulsion, there is emerging potential to apply the combined-cycle system to raise the overall thermal efficiency of commercial vessels powered by diesel and LNG piston engines.
Introduction
The most thermally efficient low-speed marine diesel engine is rated at 50 percent between fuel energy content and crankshaft power. During an earlier period of naturally aspirated diesel engines, peak efficiency of around 40 percent was common. One of the early diesel-steam combined-cycle engines dating back to 1927 was applied to railway propulsion when Kitson (UK) developed the “Still” locomotive that was briefly the most thermally efficient railway locomotive in the world.
Dating back to the early 1960’s, navies seeking the combination of high power output and high thermal efficiency combined gas turbine engines with steam power. The exhaust of the gas turbine engines were of sufficiently high temperature to convert water into saturated steam, with the addition of a small amount of heat required to produce super-heated steam that drove electrical generators.
During the early years of the 20th century, boats and ships used steam-piston and steam-turbine propulsion before the advent of marine diesel engines. Due to high the maintenance requirements of steam propulsion of the period, commercial shipping companies adopted diesel engines for propulsion as such engines required far less maintenance and much lower maintenance costs than steam propulsion.
The Energy Split
Earlier generation diesel engines that delivered up to 40 percent peak thermal efficiency released 30 percent of the thermal energy into the engine exhaust with the other 30 percent being released through the liquid cooling system. The peak efficiency only occurred over a limited range of engine speed and at maximum output, with part-load thermal efficiency dropping down to below 35 percent and over 65 percent of the energy being dissipated through the engine exhaust and cooling system. While the most efficient of modern diesel engines deliver 50 percent thermal efficiency at maximum output, part-load efficiency can drop to 40 to 45 percent.
Modern diesel engines dissipate thermal energy through the combination of the turbocharger-aftercooler, the radiator and the exhaust. In recent years, Organic Rankin Cycle (ORC) engines have been developed that circulate refrigerant compounds such as alcohol, freon, ammonia, propane and a high-pressure closed-cycle system operating on compressed carbon dioxide. In large-scale application, Ocean Thermal Energy Conversion (OTEC) uses ORC engine technology to generate power from the temperature difference of surface tropical seawater and colder seawater sourced from depths of over 6,000 feet. Heat from diesel engine radiators and turbo- aftercoolers can activate ORC-engine operation.
Diesel Engine Aftercooler
Modern diesel engines feature an aftercooler installed between the turbocharger and engine intake valves. With 32-degrees F (0-degrees C) intake air temperature, a turbocharger with 80 percent wheel efficiency and 2:1 pressure ratio will release air at 164 degrees F to the aftercooler and 189 degrees F for a 2.25:1 pressure ratio. With intake air at 80 degrees F (26 degrees C), turbocharger outlet temperatures will rise to 227 degrees F (108 degrees C) and 255 degrees F (124 degrees C) for 2:1 and 2.25:1 pressure ratios. Such temperatures are within the operational range of ORC-engines that require temperature input of 77 degrees C to 132 degrees C.
Valve timing in some engines are set to produce shorter compression stroke and longer power stroke to raise efficiency and utilize two-stage turbochargers to increase air density entering each cylinder, with 2:1 primary pressure ratio with an intercooler and 1.4:1 secondary ratio. Air entering a 4:1 pressure ratio compressor at 26 degrees C (80 degrees F) will discharge at 198 degrees C (388 degrees F). Engine exhaust at 400 degrees C (750 degrees F) entering a 2.25:1 pressure ratio turbine will discharge at 285 degrees C (545 degrees F). Both intercooler and turbine exhaust could convert water into low-grade steam.
Heat Exchangers
The installation of a pair of annular counter-flow heat exchangers on both outlets of the turbocharger would assure effective transfer of heat into piped water used to cool both the intercooler and the combustion exhaust gas. It may even be possible for the super-effective heat exchangers to condense drops of liquid water from the combustion exhaust, perhaps a sufficient volume to resupply the tanks of make-up water usually carried aboard steam-powered ships. Super-effective heat exchangers would significantly increase the density of air entering the engine cylinders prior to combustion.
Application of some design ingenuity would be required to install large-scale heat exchangers that include high-pressure water pipes, next to the engine and connected to the turbocharger. The addition of steam power would require a redesign to the ship engine room to accommodate combined-cycle operation that involves heat exchangers, super-heaters and steam turbine engine. For diesel or LNG engines under 15,000kW, a German company still offers steam piston engines rated up to 1,000 to 2,000 Horsepower (750 to 1500kw). Potential savings in fuel cost could prompt some ship companies to evaluate combined-cycle propulsion.
Steam Engine
The greatest proportion of thermal energy added to a steam power system converts liquid water into saturated steam, with comparatively little additional heat being used to raise the steam to the super-heated level. Engines with over 400 degrees C exhaust temperature entering the turbine end of the turbocharger would have sufficient heat in the combination of the intercooler-aftercooler and turbine outlet to preheat water for a steam engine. The additional input of heat required to produce high-pressure superheated steam for two or three-stage expansion, could result in a steam engine operating at 40 percent efficiency.
The combination of a diesel or LNG piston engine operating at 45 to 50 percent thermal efficiency and a steam engine recovering reject heat and operating at 35 to 40 percent efficiency relative to combustible fuel input, would yield a marine propulsion system operating at 65 percent efficiency. A steam power system that has a leak would release a benign substance into a ship while an engine that operates on ammonia or compressed carbon dioxide would represent a potentially toxic hazard to ships’ engine room personnel. The maritime industry has prior experience with steam propulsion.
Diesel Engine Cooling System
Depending on manufacturer, mega-displacement mega-horsepower marine diesel engine thermostats may be set to between 140 degrees F (60 degrees C) and 180 degrees F (82 degrees C) while smaller displacement engines may operate with thermostats set as high as 200 degrees F (93 degrees C). Closed-cycle ORC engines operating on ammonia can generate output with source temperatures down to 40 degrees C while engines that operate on freon require minimum source temperatures of 80 degrees C. In river marine service, radiator cooling system heat from a diesel engine of 1,000kW (1,340Hp) could sustain the operation of an ORC engine rated at 100kW to 120kW output.
Conclusions
The combined thermal energy leaving marine engine turbochargers with minimum of 2:1 compressor pressure ratio would be sufficiently hot to sufficiently preheat water to ease the production of steam. After being cooled in a condenser to perhaps 20 degrees C above seawater temperature, heat from the engine cooling system could preheat the water intended for steam production. It would be further heated by thermal energy from both the turbocharger aftercooler and well as turbocharger turbine exhaust, with a low pressure ratio (1.4:1) that could reduce exhaust temperature from over 400 degrees C to over 300 degrees C.
Steam leakage is much safer for engine room crew than leakage from ammonia-based or carbon dioxide-based ORC engines. With LNG engines, turbocharger exhaust outlet temperatures would still be sufficient to assist in converting liquid water into steam and engine liquid cooling systems would still provide sufficient heat for preheating water used in a companion steam engine. Steam power combined with diesel or LNG engines over 10,000kW output would raise overall energy efficiency. For smaller vessels powered by diesel or LNG engines of under 2000kW, freon-based ORC engines sourcing cooling system heat could deliver an additional 200kW of output.
The opinions expressed herein are the author's and not necessarily those of The Maritime Executive.