Super Fuel?
Can hydrogen fuel live up to its hype?
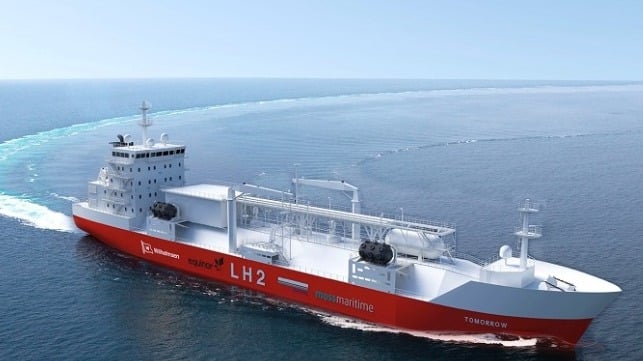
(Article originally published in Sept/Oct 2020 edition.)
Pick a color - any color - blue, green, brown or gray. Those are the colors assigned to the various forms of hydrogen depending on how it’s produced. Hydrogen has suddenly become the Superman of renewable energy. Rather than saving Metropolis, it’s needed to save the world from a damaging future created by excessive carbon emissions from burning fossil fuels.
As we’ve moved from global warming to climate change to climate crisis, the necessity of finding clean energy sources has taken on increased urgency. At one time, natural gas, which emits half the CO2 of coal, was that fuel source. At $8-$12 per million Btus, high-cost natural gas narrowed the gap with more expensive wind and solar power.
That gap widened as the shale revolution drove gas prices below $2/mmBtu. Environmentalists then switched allegiance from natural gas to hydrogen as the clean fuel savior. For the hydrogen age to begin in earnest, the fuel must overcome a number of technical and cost hurdles.
Properties
Hydrogen – the chemical element with the symbol H and atomic number 1 – is the lightest element in the universe, 14 times lighter than air, making it useful for balloons. It’s also the most abundant element, representing 75 percent of the mass of the universe. “Hydrogen” comes from the Greek “hydro,” meaning “water,” and “genes,” meaning “creator” – effectively, “born of water,” which is what is left after hydrogen is burned.
With only one proton in its nucleus, hydrogen is the smallest chemical element. It can also exist with no neutrons. Hydrogen’s small size and low density create transport and storage challenges. Given its relatively high bond strength, energy – lots of it – is required (e.g., electrolysis) to isolate hydrogen in its pure form, adding to its cost.
Hydrogen was first identified by the great experimental scientist Henry Cavendish in 1766. He produced water from hydrogen and oxygen with the help of an electric spark. The first fuel cell, which converted hydrogen and oxygen into electrical energy, was developed in 1838 by Sir William Grove using zinc and platinum electrodes. Although 20 years ahead of the emergence of the petroleum era, the discovery languished. The first commercial fuel cell wasn’t developed for nearly a century (1932).
Commercialization
Commercially, hydrogen was first used in 1792, about 25 years after its discovery, in the production of coal gas, known as “town gas.” Hydrogen accounted for nearly half the volume of town gas, which powered street lamps in the gaslight era of the 19th and early 20th centuries.
Perhaps the most amazing hydrogen prediction was its mention in Jules Verne’s 1875 adventure novel, The Mysterious Island, in which he described a world where “water will one day be employed as fuel, that hydrogen and oxygen which constitute it used singly or together will furnish an inexhaustible source of heat and light of an intensity of which coal is not capable.”
Verne was a visionary in more ways than one. The book begins during the American Civil War with five prisoners of war hijacking a hydrogen-filled observation balloon to escape. Hydrogen would become the buoyancy element that lifted Zeppelin airships and revolutionized transatlantic travel and military tactics in the decades to come.
The age of Zeppelin travel ended in 1939 with the disastrous Hindenburg accident at Lakehurst, New Jersey that killed 35 passengers in a spectacular explosion and fire and raised the question of hydrogen’s safety. Like all fossil fuels, hydrogen is highly combustible, but it requires a concentration nearly three times that of gasoline, meaning gasoline is three-times more combustible than hydrogen.
Hydrogen also disperses rapidly if a leak occurs, and its flames generate low-radiant heat in the absence of carbon, meaning they burn out quickly. Probably most important, hydrogen is non-toxic, producing only clean water when combusted in the presence of oxygen, anointing it as the fuel to power the carbon-free economy of the future.
Adding to its desirability is its versatility. As a gas, it can both utilize the existing natural gas pipeline infrastructure and power electricity-generating turbines. In liquid form, it can be used as a transportation fuel, which is the energy sector’s most bedeviling clean-energy solution. Electrifying the globe’s vehicle fleet creates environmental issues from rare earth mining to battery disposal. As a fuel that can be produced while eliminating or minimizing carbon emissions, hydrogen is much more desirable.
The Color of Clean
The four versions of hydrogen are colored by the carbon volumes emitted during production. Brown hydrogen is made from coal while grey hydrogen comes from natural gas. In both cases, the CO2 from the production process is emitted into the atmosphere – more with coal, less with gas.
In contrast, blue hydrogen is derived from natural gas with the emissions captured and stored. The ideal hydrogen is green, which is produced from renewable fuels, leaving water as the lone emission.
Europe is finding that its aggressive push into clean energy has resulted in significant surplus wind and solar electricity being produced when it’s not needed, forcing utilities to pay producers for the wasted power. The intermittency of wind and solar power means that generating capacity two-to-three times the required power has to be built, resulting in an investment misallocation. That misallocation is magnified by the fact that these intermittent power sources have operating lives of only 15-25 years, compared to fossil fuel power plants with 40-60 year lives.
The Cost Challenge
The greatest hurdle for hydrogen is the cost of production, especially if it must be green. A study by the Hydrogen Council determined that, using an import price of $3 per kilogram of hydrogen to power turbines, the electricity produced would cost about $140 per megawatt-hour (MWh).
By comparison, a 2019 estimate of electricity’s levelized cost suggests the unsubsidized cost of natural gas combined-cycle electricity generation is between $44/MWh and $68/MWh, a current 50 to 70 percent cost advantage compared to the hypothetical hydrogen-generated figure. If the hydrogen import price could be reduced to $1 per kilogram, the resulting electricity would be price-competitive with electricity produced from natural gas with the added benefit of no carbon emissions.
Attacking the cost challenge is motivating companies to experiment with projects to produce green hydrogen with cheap renewable electricity. These experiments are targeting offshore wind and solar installations to manufacture hydrogen using surplus electricity. The hydrogen would be stored and then utilized to generate electricity when the renewable power sources are unable to supply power.
Alternatively, these installations could produce hydrogen at a reduced cost by using the wasted electricity and injecting it into the continent’s gas pipeline network. It could also be delivered in liquid form to power the transportation sector. There are plans to create a network of liquid hydrocarbon storage and fueling facilities along the major rivers of Europe that would power fuel-cell equipped barges and ships, reducing carbon emissions. A fuel-cell vehicle market could also be in Europe’s future, such as exists in Southern California.
The attraction of hydrogen for transportation is its high energy density, albeit with certain caveats. In particular, hydrogen has a high energy content relative to other fuels per unit of weight, but a lower energy density per unit of volume.
Hydrogen has three times the energy content of gasoline, but on a volume basis that relationship is reversed. A fuel-cell vehicle needs to be powered with high-pressure hydrogen that will require high-strength storage tanks made of reinforced composites, adding to a vehicle’s cost.
Importantly, hydrogen has a higher energy storage density relative to lithium-ion batteries. One kilogram of hydrogen has the same electrical energy as one gallon of gasoline. With the tank-to-wheel efficiency of a fuel cell vehicle twice that of an internal combustion engine vehicle, one kilogram of hydrogen provides as much range as two gallons of gasoline. With falling hydrogen prices, fuel cell vehicles would become very competitive, especially by having fewer environmental issues than battery-powered vehicles.
Unanswered Questions
Besides the cost issue, there are unanswered questions about storing and transporting hydrogen. Its small molecules enable it to permeate steel pipes at a rate four-to-five times that of methane, causing embrittlement and necessitating gas pipes be replaced, as well as appliances if hydrogen is used.
Natural gas storage uses either salt domes, aquifers or depleted oil/gas reservoirs. In Europe, only salt domes have been examined for hydrogen storage, so questions remain as to whether sufficient capacity exists. Alternative storage options will need to be investigated, or possibly additional salt dome storage will need to be constructed. Until hydrogen is proven to be a commercially viable fuel, storage at scale is not a restraint.
Europe is investing heavily in wind and solar power to meet its clean energy goals. Every energy study, however, points to its inability to meet those targets without hydrogen playing an important role. Recently, Europe has experienced low wind and solar output, magnifying utilities’ challenges to deliver electricity on demand.
Everyone is betting heavily on dispatchable hydrogen’s commerciality, but it’s unknown when – or if – hydrogen’s economic and technical challenges can be overcome. If not, green energy plans face a day of reckoning.
The opinions expressed herein are the author's and not necessarily those of The Maritime Executive.