Revisiting Vanadium Flow Batteries for Ship Propulsion
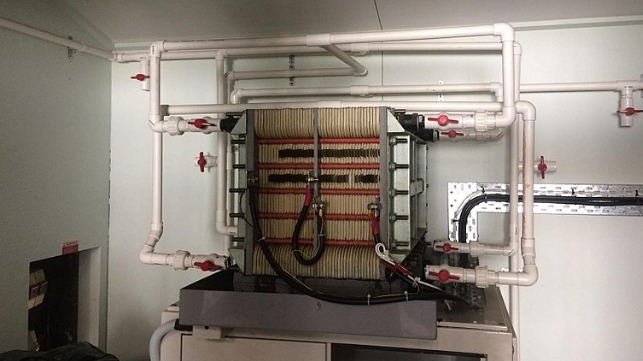
In July 2019, Maritime Executive carried a commentary suggesting possible application of vanadium flow batteries for vessel propulsion. More recently, companies from Germany and the Netherlands have expressed in further developing vanadium flow battery technology for large vehicle propulsion applications. Their interest offers the possibility of an expanded system involving several modes of large transportation vehicles.
Introduction
The history of electric propulsion begins with Werner von Siemens development of the electric tram or streetcar that drew power from an overhead power cable and replaced horse-drawn trams in municipal passenger transportation service. At the time parallel development in electric storage battery technology led to the appearance of battery-electric vehicles. However, development of competing energy storage and propulsion technologies led to the demise of battery-electric road vehicles. The combination of thermal power stations and hydroelectric power generation sustained the operation of electric municipal public transportation systems and electric railway lines.
In more recent years, emergence of power generation technologies such as nuclear power, solar power conversion, advances in wind energy conversion along with ocean energy conversion have increased the need for grid-scale energy storage capability. Ongoing concerns about gaseous emissions into the atmosphere have prompted re-examination of battery powered transportation technologies, courtesy of ongoing advances in electrical energy storage technology. One of the advances involves the flow battery where energy is stored in liquid electrolytes. Such technology offers greatly extended useable deep-cycle discharge life expectancy, with vanadium flow batteries being one of the promising energy storage variants.
Mobile Batteries
During the 20th century, lead-acid batteries formed the basis of electric battery car propulsion. E-bikes and e-scooters comprise the most widely used electric passenger vehicles in urban environments, with the majority using lead-acid batteries that offer a repeated deep-discharge life expectancy of around 300-cycles. While lithium-ion batteries offer much higher energy storage density, the useable life expectancy depends of the depth of repeated discharges. Carbon-foam batteries use the same sulfuric acid as lead-acid batteries, with energy density approaching lithium-ion batteries and 3,500-cycles when repeatedly discharged to 50 percent. Several grid scale batteries offer up to 20,000 deep-discharge cycles.
While originally developed for stationary application, flow redox batteries can be adapted for large-scale vehicle propulsion. Vanadium redox flow batteries (VRFB) store electrical energy in pairs of large tanks containing liquid electrolyte. Researchers at Australia’s University of New South Wales developed improved electrolytic compounds of vanadium-bromide and vanadium chloride that are stored inside separate tanks, offering some 50-watt-hours/liter or 100 percent increase in the energy storage capacity over earlier VRFB’s. Repeatedly deep discharged from 100 percent to 5 percent of its maximum capacity, the technology can deliver up to 20,000-cycles of useable power in both stationary and mobile application such as ship propulsion.
Ship Energy Requirements
A ship requiring 25MW of power to sail at a speed of 25-knots would require less than half that power of 5.5MW to sail at a speed of 15knots, requiring almost 30-hours to sail a distance of 500-miles, or 165MW-hr of power. Compensating for electric motor and propeller efficiencies would require 240MW-hr of power. A pair of storage tanks each holding 5,000-cubic meters of electrolyte would provide 250MW-hr of the required energy. The greatly extending deep-discharge cycle capability of the flow battery technology enhances its competitiveness against other batter technologies such as lithium-ion.
When the electrolyte has reached its expected useable life expectancy of 20,000-deep-cycle discharges, it may be possible to extract pure vanadium from the liquid electrolyte at low cost, subsequent to which there would be a variety of market applications for the vanadium. That vanadium could be used in the manufacture of future alloys or be processed for another 20,000-deep-cycle use in future redox flow batteries.
Sailing Routes
The space aboard large ships allows the total combined electrolyte storage tank capacity to increase to 20,000 and even 30,000-cubic meters, greatly increasing ship sailing distance. Battery electric ships with smaller tanks could cover routes across the Baltic Sea, radiating from Stockholm to Tallinn, Helsinki and Gdansk, also Helsinki – Gdansk. Mediterranean routes would include Tunis – Rome, Tunis – Marseilles, Valencia – Marseilles, Rome – Barcelona and Genoa – Barcelona. Such ships could sail across the North Sea from Edinburgh to Goteborg, Hamburg or Rotterdam. Suitable South American routes would include Buenos Aires – Montevideo and Rio de Janeiro – Santos (Sao Paulo).
Possible South Pacific routes would include Christchurch – Wellington and Hobart – Sydney. Routes extending from Shanghai would include Incheon (Seoul), Busan, Nagasaki and Taipei. Ships with larger electrolyte storage tanks would be able to operate from Singapore to either Jakarta or Ho Chi Min City and also cover the distance between Hong Kong and Manila. Such ships would also be able to sail Lubeck – Helsinki and extended Mediterranean routes such as Rome – Valencia, Genoa – Valencia, Tunis – Marseilles and Tunis – Barcelona. Battery ships could sail from Ports of Algeciras and Tangier to Lisbon, Oporto, Algiers, Valencia and Barcelona.
Port Energy Storage
The ability of flow batteries to store electrical energy in an electrolytic liquid allows for large tanks to store such liquid at ports. When a ship arrives at port for a short stopover, spent electrolytic fluid may be pumped into port-based recharge tanks with ship onboard storage tanks subsequently refilled with recharged electrolyte. During an extended stopover, the electrolytes would be recharged courtesy of an electrical connection to the local power grid. Port storage of electrolytic fluid allows for VRFB technology to provide propulsive power for other large vehicles that either visit the port or operate within the port area.
Railway Propulsion
Trains travel to and from maritime ports, usually carrying bulk or container freight and sometimes carry passengers (tourists). During an earlier era, steam railway locomotives pulled a semi-permanently coupled fuel tender unit that carried coal and water. A locomotive chassis adapted to VRFB technology would include a tender unit built with a pair of large tanks, one tank carrying vanadium-bromide and the other carrying vanadium-chloride. Special flexible pipes that connect between locomotive and tender unit would transfer electrolyte to battery stacks housed within the locomotive and that produce electric power for propulsion.
Depending on the weight of the train and train speed, VRFB propulsion could provide anywhere from 100-miles (160-km) to perhaps as much as 300-miles (500-kms) operating distance. There would be scope to develop VRFB locomotives for multiple-unit operation to move heavier trains greater distance at higher speed. During the steam locomotive era, the Garret locomotive comprised water tanker sections couple ahead and behind the locomotive. A comparable layout for a VRFB locomotive would involve electrolytic solutions being carried in the forward and trailing tanks of almost 200-cubic meters each, containing 10MW-hrs of energy to allow for extended distance operation.
Trucks
The configuration of a truck converted to VRFB propulsion would be based on that of a fuel tanker delivery truck, with the driver’s cab positioned above the front axle(s). A separate compartment located behind the driver’s cab would house the battery stacks, with a pair of tanks of 2,000-litres each secured side-by-side above the chassis and ahead of the articulation coupling. The tanks would hold 100kW-hrs of energy, perhaps making VRFB trucks suitable for operation within port areas or operation over short distances to destinations located within the same metropolitan region near the port.
Energy Sources
While the perceived need to reduce vehicle engine exhaust emissions propelled development of battery-electric transportation vehicles, such vehicles have access to competitively priced electrical energy during the overnight hours, in many locations internationally. Season flooding may often require overnight operation of hydroelectric power dams and the need to main system reliability results in many thermal power stations being operated at near constant power output, with excess off-peak power being transferred into grid-scale energy storage technology. Battery-electric vehicles and energy storage systems that can be recharged during the overnight off-peak hours represent business opportunities for power companies.
Conclusions
Large-scale battery-electric vehicles such as ships, ferry vessels and railway locomotives require competitively-priced battery technology capable of enduring 20,000-repeated deep-cycle discharges of up to 95 percent of storage capacity. Liquid metal battery, flow battery and liquid air storage technology are among the very few potentially mobile electrical energy storage technologies capable of achieving such levels of performance. At the present time, companies developing vanadium redox flow battery (VRFB) technology have a head start over their competitors in terms of offering an energy storage technology capable of providing cost-competitive distance and endurance performance.
The opinions expressed herein are the author's and not necessarily those of The Maritime Executive.