Global eNav - Great Benefits Awaiting a Resilient System
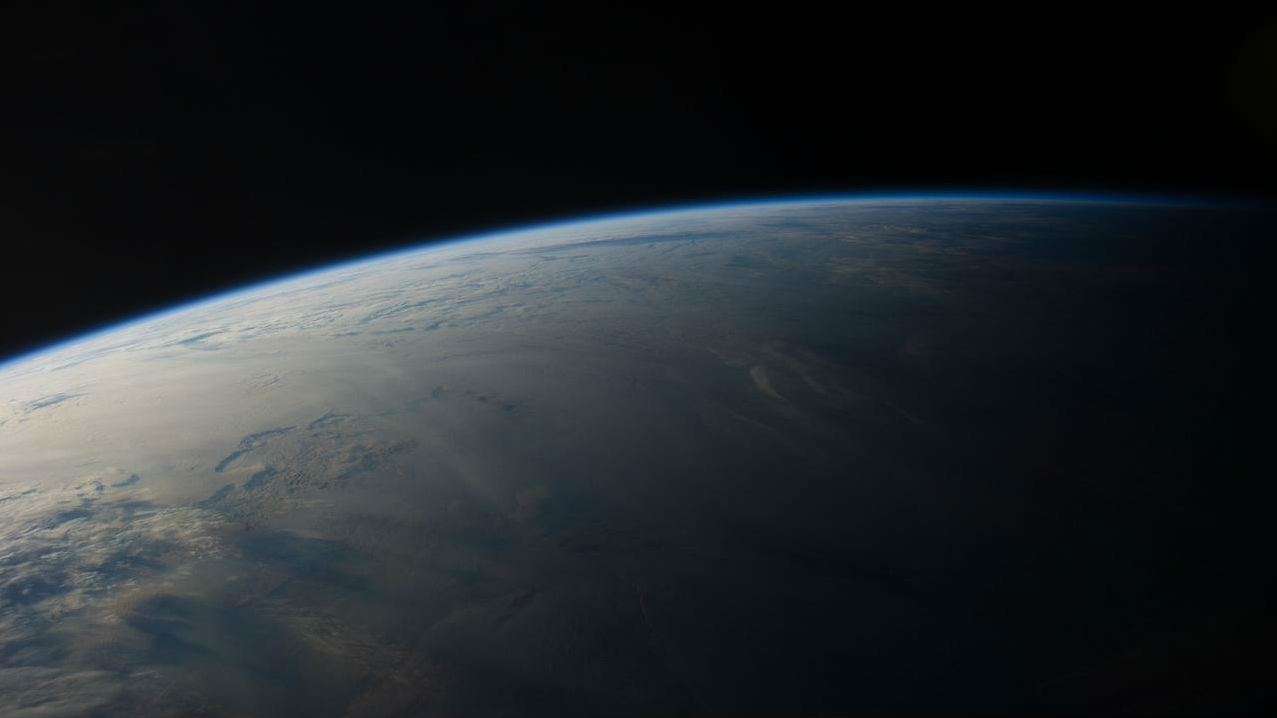
The next great leaps for international maritime productivity are poised to be the introduction of autonomous/remotely operated vessels, and the multitude of maritime and supply chain efficiencies brought about by the promised but long-delayed implementation of IMO’s eNavigation concept.
Unfortunately, both opportunities rely upon a resilient electronic positioning and navigation architecture that IMO first called for in 2008, but which still does not exist.
The world has abundant electronic navigation, of course, from satellites like the Global Positioning System (GPS), and it is now the primary means by which mariners navigate. However, satellites alone are unable to provide IMO’s “…robust, reliable and dependable” signals for safety of navigation and safety of life applications.
Global Navigation Satellite Systems (GNSS) do continue to improve and grow in number, as discussed below. These upgrades will provide marginal increases in robustness and reliability for those equipped to access the systems. Nevertheless, all GNSS transmit very faint signals in the same general frequency band. These can easily be disrupted by natural phenomena, malicious actors and even system failures. There is ample evidence that local disruptions are happening all the time.
Successful implementation of autonomous/remotely operated vessels and/or eNavigation will require that this fundamental shortfall be addressed. GNSS must be paired with another source or sources such that the combined systems are virtually impossible to disrupt.
The following is a summary of proposed and on-going projects that will help improve electronic navigation, and in some cases could make it bulletproof:
Global Navigation Satellite Systems (GNSS)/ Regional Navigation Satellite Systems (RNSS)
GNSS signals are highly precise and free for use almost anywhere with a view of the sky. They should be used whenever services are available and can be trusted. Two independent constellations are currently deployed and two more are in the process. Even greater location precision can be had by accessing Space Based Augmentation Systems (SBAS), such as WAAS, EGNOS and MSAS, and Ground based Augmentation Systems (GBAS). The IALA maritime radiobeacon DGPS service, which augments and improves the precision for GNSS in various regions of the world, is one example of a GBAS.
As mentioned earlier, additional satellites alone will not create the needed resilience, but accessing all satellite systems and improvements to the satellites and their signals will marginally improve performance and reliability for properly equipped users.
Satellite navigation receivers must be specifically equipped and configured to access and use more than one of the constellations listed below. Basic services from all the government-sponsored satellite navigation systems are available for use without charge.
GPS, United States (global coverage) – The Global Positioning System has long been considered by the United States as “a gift to the world” with its pledge of making signals freely available to all. GPS satellite and ground control systems have refreshment projects underway. Additional frequencies are planned, along with a more secure capability for use by US military forces.
GLONASS, Russian Federation (global coverage) – Russia’s “GLObal NAvigation Satellite System” first became operational in 1993. Since that time, funding and technical problems have occasionally impacted the usefulness of the system. As of this writing, the system is generally considered operational and effective. The Russian Federation has a deliberate maintenance and continual upgrade plan in place to ensure continued success.
Galileo, European Union (global coverage)– This system has 18 satellites in orbit and its operators say they will declare the system operational (users will be able to navigate using just Galileo satellites) when a total of 24 satellites are available for use. Properly equipped receivers are already able to use existing Galileo satellites in conjunction with those from other systems to safely obtain and compute location.
BeiDou, China (global coverage) - Scheduled to reach “full operational capability” in 2020, BeiDou has 17 usable satellites in space. Properly equipped receivers are already able to use existing BeiDou satellites in conjunction with those from other systems to safely compute location.
QZSS, Japan (Asia & Oceania) – This regional system will consist of four satellites when it is operational in 2018. It is designed to augment GPS and enable very high precision positioning.
IRNSS/NAVIC, India (India & vicinity) - The India Regional Navigation Satellite System will have seven satellites and was expected to be operational in 2018. As with the Japanese system, it is designed for very high precision applications. The operational date may now be set back by the recent launch failure of a component satellite.
Commercial Satellite Systems
Satelles (coverage based on subscriber demand) – Using low earth orbit Iridium satellites, Satelles uses stronger signals that the national systems. It makes these available to subscribers for a fee. The company says that it can provide services anywhere on the globe to an unlimited number of subscribers. However, analysis of the performance of the system against the four required navigation performance (RNP) parameters, accuracy, availability, integrity and continuity, in the maritime domain, has yet to be performed.
Loran-C/eLoran
Loran is a terrestrial radionavigation system that was first developed in in the mid 20th century. The technology has been continually improved, with the most recent versions referred to as Loran-C and eLoran. With a terrestrial, low frequency, high power signal, Loran is very difficult to disrupt and is complementary to GNSS.
Loran-C signals are available in most Russian, Chinese, Saudi Arabian and South Korean navigable waters. India has Loran-C along its northwest and northeast coasts.
eLoran is a more accurate, and robust state-of-the-art version of Loran. It was implemented as a test-bed at Initial Operational Capability (IOC) level (providing 10m (95 percent) for harbor entrance and approach (HEA) applications), for use along the east coast of the United Kingdom for 12 months. Its use as one component of a multi-system receiver was demonstrated as part of the Resilient-PNT stream of the European Union’s ACCSEAS project. Experts in the U.S. have recently opined that a receiver combining GPS satellite and eLoran signals would be virtually impossible to disrupt. The United States government has twice announced that it will build an eLoran system for use by maritime, other transportation modes, and critical infrastructure. The U.S. Congress is actively considering legislation this year to mandate establishment of the system and fund a $10 million proof of concept project starting on October 1, 2017.
Loran systems, at present, are typically effective to 1,000 NM. This could improve with further developments in receiver and transmitter technology.
R-Mode
R-Mode is the addition of a ranging signal to other maritime radio signals. Two candidate systems are being investigated: the IALA Medium Frequency (MF) differential-DGP service, which operates in the marine radiobeacon band at 285 kHz to 315 kHz; and the Automatic Identification System (AIS), which operates in the marine VHF band at around 162 MHz.
The work is being driven by the maritime administrations of Germany and the Netherlands and is based on the principle of the re-use of existing MF DGPS beacon transmitter infrastructure. Ranging trials have demonstrated an accuracy of 3-4m (1-?). There is a marked difference in performance experienced between day and night because of the prevalence of skywave interference at nighttime.
Dalian University in China has demonstrated AIS R-Mode over a short range, achieving 10m positioning accuracy in areas with good transmitter geometry. The geographical range of an MF broadcast is around 150 to 200 kilometers, while that of AIS is approximately 50 kilometers line of sight. It is planned that work on R-Mode will continue in Europe under the auspices of the R-Mode BALTIC project, which starts in September this year. Also, the General Lighthouse Authorities in the U.K. and Ireland are undertaking a project this year looking specifically at AIS R-Mode.
Radar Absolute Positioning
A number of techniques for absolute radar position fixing have been investigated. This is a technique of deriving latitude and longitude (also course and speed over ground) from radar returns. Previous trials, performed under the ACCSEAS project using active transponders (eRacons) with a modified radar were very successful in demonstrating sub 10m positioning accuracy within 10 NM of the coastline.
The usefulness of the technique is dependent on a large amount of expensive and high maintenance, shoreside infrastructure. In addition to their high initial capital outlay (~$30k), eRacons need power, monitoring and physical security. There are techniques however that rely less on expensive shoreside infrastructure and more on passive radar returns employing map/feature mapping and tracking techniques. Some of these techniques look very promising, with one in particular (RadarFix) having been in operation in Newfoundland over 20 years ago, purportedly demonstrating positioning accuracies of approximately 2m. The General Lighthouse Authorities of U.K. and Ireland have been investigating the independent development of their own radar positioning algorithm, which has some resemblance to techniques found in the literature and practice.
It is believed that radar-return images are unique enough that an automated image-recognition algorithm would be able to associate a particular radar image with a geographical location. Recent trials at the General Lighthouse Authorities of U.K and Ireland have demonstrated the usefulness of integrating radar map matching with doppler speed log and gyro-compass in a method called Radar Dead Reckoning. Such techniques allow resolution of geographical ambiguities in radar imagery and smoothing during the time between the epochs of radar image capture. Accuracies in the region of 15m (95 percent) have been demonstrated, post-mission, in the Harwich and Felixstowe harbor area.
Signals of Opportunity
Methods that employ transmitted signals whose primary purpose is for some other application unrelated to navigation are referred to as Signals of Opportunity techniques (S-Op). The most promising signals of opportunity appear to be DAB radio and DVB-T (digital terrestrial television) broadcasts.
Components of both signals are broadcast with a pseudo-random bit sequence (PRBS) that is known by the receiver in advance. These ‘pilot’ signals are broadcast to aid receiver tracking and demodulation of the audio / video stream. Correlation of the pilot data with a local replica generated by the S-Op receiver can provide an accurate pseudo-range measurement. Accuracy of the system will be limited by multi-path interference and the limited geometry of available transmitters. As a bonus, the network must be precisely time-synchronized to operate as a Single Frequency Network (SFN) so all digital transmitting stations have precise GPS-synchronized clocks. Because of this last point, any resilience created by the use of such S-Op techniques needs to be carefully assessed.
Bathymetric Navigation
Sonar can be used to perform terrain referenced navigation (TRN), or bathymetry, underwater. A multibeam sonar echo-sounder is used to measure the range from the host vessel to a number of points on the seabed. The relative positions of these points, obtained from the sonar measurements, is known as a bathymetric profile. By matching this with a database, the vessel’s position may be inferred. This is sometimes known as bottom-contour navigation (BCN).
The performance depends on the terrain height variation and sensor resolution. The highest resolution sensors can measure more than 10,000 points simultaneously. The positioning accuracy can be as good as 1m but is typically around 10m. This technique is typically deployed on underwater vehicles. Surface vessel positioning may be less precise because the sensor will be further away from the sea bed, though noise-like errors may be smoothed out through integration with dead reckoning.
Onboard Systems
Other than over intervals of a few minutes, inertial navigation performance is significantly poorer than that achievable using traditional dead reckoning with ground velocity measurements. Currently the most capable inertial system available for use aboard ship is very expensive and even then, its accuracy
degrades rapidly once calibration by GPS is lost. Stand-alone inertial navigation is not currently recommended as a reversionary mode as its performance is inferior to that of a gyrocompass and Doppler Velocity Log. The use of Doppler speed log and gyro-compass provides greater positioning accuracy for longer periods of holdover time once GPS has been lost to jamming or interference or other system failure.
Conclusion
A recent article in the industry journal GPS World concluded that eLoran is the only complementary backup system that can be implemented within the timescale envisaged for the introduction of e-navigation; however, there are political obstacles to implementation, at least in Europe.
Dr Brad Parkinson, widely known as the “Father of GPS,” has opined that the ideal navigation suite includes what he calls the “resilience triad,” – GNSS, eLoran, and an inertial system. The authors of this article heartily agree.
We also agree that the small marginal cost for robust and resilient navigation will be repaid many times over by the substantial increases in productivity and profitability that will ultimately be realized.
Acknowledgements: The authors thank, Dr Alan Grant, Martin Bransby and Martin Faga for their review of and contributions to this article.
Captain Dana A. Goward is retired from the US Coast Guard and the President of the Resilient Navigation and Timing Foundation.
Dr Paul Williams is Principal Development Engineer at General Lighthouse Authorities of the U.K. and Ireland.
The opinions expressed herein are the author's and not necessarily those of The Maritime Executive.